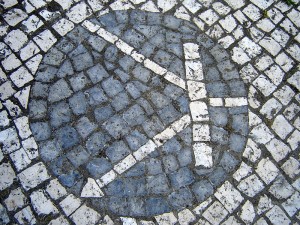
In part 1 of this series, I discussed the four laws of thermodynamics. These laws tells us that the total change in energy in a system, dU, is composed of two parts:
dU = dQ – dW
where dQ is the heat energy added to the system and dW is the work done by the system. The Earth is such a system, and one not isolated from external heat sources (the Sun). In part 2, we learned how atmospheric gases like water and CO2 trap heat near the Earth’s surface and prevent the Earth from re-radiating all energy received from the sun back out into space. This allows the surface of the Earth to remain at a temperature hospitable to life as we know it. That temperature is slowly increasing over time.
But in part 2, we also observed that CO2 is an inferior greenhouse gas when compared to water, which has a higher overall contribution to the atmosphere. What is the relationship between CO2 and Water in the climate disruption cycle?
Consider a transistor. Don’t know what that is? No problem – it’s pretty easy to explain. A transistor is a device that allows a large electric current to flow at the pleasure of a much smaller electric current, called the “base” current. The base current can be provided by a very low energy source. This allows for control of the much larger current. Being able to control a large current with a much more modest one is the basis of electric amplifiers. Such devices take a weak signal, such as the crummy sound from your tinny iPhone speaker, and instead use the weak signal to control a much stronger electric current. That larger current drives more energy through bigger speakers, and the result is that your puny little iPhone audio output can be AMPLIFIED by regulating a much larger current that drives more power through much bigger speakers. And voila! You have a dance party.
Weak signals that cause changes in strong signals can have a profound impact. Let’s consider that same principle applied to water vapor and CO2 in the Earth’s atmosphere.
The amount of water in the air, especially closer to the surface of the earth, varies a great deal over the course of a day or a season [1]. In a dry climate where there is little water vapor to evaporate into the air during the course of a day, the night will grow very cold very fast because there is so much less heat-trapping water vapor in the air. In a moist climate, humidity will trap heat at night and maintain a relatively high temperature, closer to the daytime temperature. We already have a lot of experience with the effect that water vapor has on a local climate.
Water changes phase very readily in response to the typical pressure and temperature conditions near the Earth’s surface. Condensation of water releases the heat of vaporization originally input the get the water to the gaseous state; this release of energy during condensation in a primary driving mechanism in placing large amounts of energy into the atmosphere, powering weather phenomena like storms. The freezing of liquid water in the atmosphere leads to formation of clouds. Gases like CO2 and CH4 (methane), however, DO NOT change phase readily during normal Earth pressure and temperature conditions; as a result, they remain in gaseous form, and thus in the atmosphere, unless taken up by biomass on the surface of the Earth or chemically converted in the air. Water, on the other hand, doesn’t remain in the gaseous state very long; water’s life cycle in the atmosphere is short – about 9 days, on average. By comparison, CO2 and CH4 can remain in the atmosphere for decades, even centuries.
So what is the amplifier here? We see that water, while a powerful greenhouse gas, has a volatility in the atmosphere controlled primarily by the local variations in temperature and pressure over the course of a day or a season. CO2 and CH4, also greenhouse gases, remain as gases and thus in the atmosphere unless consumed by biomass or other means. CO2 and CH4 are the “weak signals” – they don’t trap a lot of heat, but they do trap heat. More of these weak greenhouse gases traps a little more heat.
More heat causes more water to enter the gaseous phase as temperature increases. Adding a little more water to the atmosphere leads to a great deal more heat being trapped. While water vapor concentrations vary over the course of a single day or a season, as the average amount of CO2 and CH4 increases so does the average amount of trapped heat, and thus the average amount of water vapor concentration also trends upward. A small change in CO2 or CH4, trapping only a little more heat, can lead to more water vapor, which traps a lot more heat.
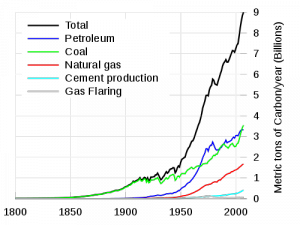
Prior to the industrial revolution, the concentration of CO2 in the atmosphere was between 260-280 ppm (parts-per-million). Measurements from Antarctic ice cores show that this range was steady for the 10,000 years preceding the industrial revolution. After the industrial revolution, the consumption of fossil fuels as an energy source began to introduce increasing amounts of carbon compounds, including CO2, into the atmosphere. See the graphic at the left, from the Department of Energy [2]. Since the industrial revolution, CO2 concentrations have increased from 280ppm to 380ppm. Half of that increase, 5o ppm, took place after 1973.
What about water vapor? Measurements of specific humidity (the ratio of water vapor to dry air in a volume of air) suggest that water has increased in concentration in air, globally, by about 0.1-0.2 g/kg-1. The data I was able to find go back to about 1975 [3]. This paper uses surface observations (on land and at sea). Other observations, such as satellite measurements of moisture content, confirm a similar trend.
So there is evidence that in our climate system, more trapped heat is leading to higher concentrations of water. The Clausius-Clapeyron relation predicts this behavior – hotter air can hold more water vapor. But water has a short life cycle in the atmosphere, condensing back to Earth as water (dew, rain) or freezing the winter into ice or snow. CO2 and other gases don’t get to do that in the Earth climate; it’s too hot, even at freezing temperatures, to condense CO2 and CH4 in liquid form. They remain in the atmosphere, free to trap more heat continuously over time.
So water is a positive-feedback mechanism for warming. A little more CO2 traps a little more heat, which puts a little more water into the atmosphere during the hottest part of the day. This traps even more heat. Water condenses out of the atmosphere at night, while CO2 remains, and the whole thing repeats the next day. Over time, less and less heat is able to radiate away from the Earth.
The consequences of this behavior on small regional scales are not completely predicted. But the cycle is there, and it begins with carbon. Breaking that cycle will take policy initiatives, not poor education. The greenhouse effect has made our planet hospitable to life as we know it. It is an inevitable by-product of the laws of physics and the chemical properties of elements and molecules. Our carbon feast, a by-product of our energy needs, feeds the greenhouse effect and increases its potency consistently over time. None of this is in dispute in the scientific community.
We all want easy answers to hard problems. Students in school just want to know the answer to problem 5, without any concern for how to solve the problem or what the answer means. When a tragedy occurs we seek to pour blame on a single cause, a single actor. We want easy answers in a complex world. But sometimes, problems like that of CO2 require us to execute a shared sacrifice alongside a difficult set of technological innovations. It’s doesn’t matter that we want a simpler answer; it matters that we have the courage to accept the true answer.
[1] http://en.wikipedia.org/wiki/Water_vapor_feedback#Role_of_water_vapor
[2] http://en.wikipedia.org/wiki/Fossil_fuel
[3] Dai, Aiguo. “Recent Climatology, Variability, and Trends in Global Surface Humidity.” Journal of Climate, August 2006. http://www.cgd.ucar.edu/cas/adai/papers/Dai_JC06-sfcHumidity.pdf