The Nobel Prize committee planned the announcement of the 2017 prize in Physics for Tuesday, October 3, at 11:45am CET (4:45am US Central time). I got up early this morning to connect to the live stream and listen to the announcement. The Nobel Committee announced that this year’s prize goes to Rainer Weiss (one half), Barry Barish and Kip Thorne (splitting the other half) for decisive contributions to the LIGO Detector and the observation of gravitational waves [1].
In this short post, I reflect on the discovery of gravitational waves by direct observation, a measurement over a century in the making.
Ever used GPS to navigate to an unfamiliar location? Then you have used Einstein’s General Theory of Relativity. Without it, the calculations needed to coordinate satellite data in your GPS – be it on a mobile phone, dedicated navigation system, or your sports smart-watch – would be more and more wrong by 11km (6.8 miles) every day. This is due to two effects: the different way time passes on Earth and in orbit, and the speed of the GPS satellites relative to the surface of the Earth. [2]
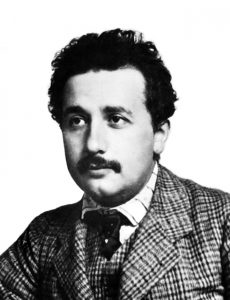
Don’t worry if you’ve never heard of the General Theory of Relativity. While it underpins our modern understanding of the Universe, including day-to-day things like GPS-based navigation, it’s also one of the hardest-to-grasp ideas that sprang from the life, work, and mind of Albert Einstein. When he was just 26 years old, a few years after completing a teaching diploma and just after finishing his PhD, he published four seminal papers (1905) that eventually catapulted him into the beginning of a highly visible career in physics. In two of his 1905 papers, he laid the groundwork for his later much bigger discovery, the General Theory of Relativity. He showed how the relativity of measurement of space and time due to motion, and the invariance of the speed of light under changes of state of motion, could explain many of the puzzles left over from the previous great era of physics exploration: Newtonian Mechanics, Electricity and Magnetism, and Thermodynamics.
Over the next decade, Einstein developed a much deeper idea – that gravity was connected in some way to space and time. His goal was to accomplish what Isaac Newton and Galileo Galilei had been unable(despite lifetimes devoted to scientific investigation) to do : explain the nature of gravity. Galileo showed how falling in a gravitational field is not affected by mass, but could make no more sense of it. Newton determined the form of the law of gravitation between any two masses, but could find no evidence to explain why gravitational forces were attractive, why they could act over distances without physical contact, and why the law of gravitation took its particular form.
Einstein came to recognize, through the application of complex mathematics (especially geometries of curved spaces), that mass and energy cause space and time to bend and warp; and that the bending and warping of space and time affects the motion of matter and light. This is the nature of gravity, and explains everything that Galileo, Newton, and others had observed or calculated about this force. Einstein’s own idea was powerful on its own because it predicted new phenomena that had not (yet) been observed. One of these was gravitational waves.
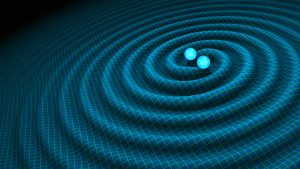
What are gravitational waves? In short, they are ripples in space and time. General Relativity predicts they travel at the speed of light. They can be generated by the motion of masses about one another, but to be detectable the masses that cause them have to be huge… immense… the most extreme masses in the Universe.
To date, the only things in the universe that will do are the corpses of collapsed stars. A single corpse will not do. Rather, one needs at least a pair, bound to one another by gravity and orbiting in a cataclysmic dance that ends in their union. Stars, if they are heavy enough, will die in one of two spectacular ways: they will collapse, either under their own mass or by accumulating mass from a partner star, into an extreme object called a neutron star; or, if even heavier, then collapse past the neutron star stage into a more extreme object called a “black hole.” Neutron stars and black holes can be home to many times the mass of the Sun, and the biggest black holes that are known contain the mass of hundreds of millions of suns.
When these extreme corpses dance about one another, they so distort space and time that the distortions travel away, carrying energy with them, in the form of gravitational waves. By the time they reach earth, these ripples in space and time are just a tiny fraction of an atom in size. To detect them, you need a sensitive and very large instrument. You need a gravitational wave observatory.
The quest to detect these waves culminated in 1997 in the formation of a scientific collaboration called the LIGO Scientific Collaboration. “LIGO” stands for “Laser Interferometer Gravitational Observatory.” These devices use intense, identical beams of light sent on two different paths. They are made to travel those paths many times, and then the light is recombined. If either of the paths were distorted as a gravitational wave passed through the Earth, one of the two paths will shorten or lengthen compared to the other. This results in a distortion of the overlapping laser beams that can be seen by sensitive instrumentation. It’s actually a simple idea, but to create an instrument precise enough to detect sub-nuclear distortions of space and time required the technological advances in the 80 years after Einstein formulated his gravitational wave predictions. It simply was not possible to make the necessary instrument earlier.
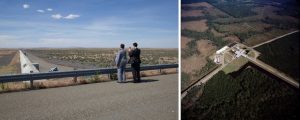
The LIGO Scientific Collaboration was formed to build and operate such an instrument. LIGO was constructed at two sites in the United States: Hanford, Washington and Livingston, Lousiana. Two instruments meant that signals in one could be confirmed in the other; and, more importantly, the prediction that gravitational waves travel at the speed of light could be tested by measuring how long such waves take to travel between the two sites.
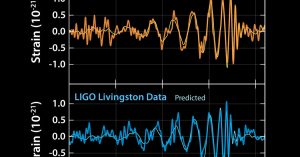
By 2015, LIGO had been through multiple phases and had entered the new “Advanced LIGO” phase. Advanced LIGO represented a leap in the original design of these instruments, and in the autumn of 2015 the collaboration was operating them in an engineering run – a shakedown before doing a “science run,” intended to collect publication-quality data. But nature doesn’t care what run you are in; if nature sends a signal, you had better be listening. Indeed, in September of 2015 the collaboration detected a signal at both sites. After a tremendous amount of work to verify the signals were not false positives, the collaboration announced the detection of gravitational waves on February 11, 2016. They had detected the death spiral of a pair of huge black holes – each with masses in excess of 25 times the mass of the Sun – merging into a single black hole and radiating off enough energy in gravitational waves to have made three of our Suns. This was an incredible death, heard more than a billion years after it happens, that shook space and time itself.
Later in 2016, LIGO reported evidence for a second such merger of two black holes. This one had been recorded in 2016, and though the black holes were smaller in mass the detection was still considered strong. The fact that in just 6 months these two detectors could hear the death spirals of two pairs of huge black holes suggests such events might be common in the Universe. These observations also usher in the era of gravitational wave astronomy, when the instruments go from being science projects to reliable tools for making measurements of the Universe.
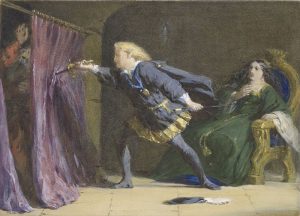
Imagine that the Universe is the play, “Hamlet,” by William Shakespeare. For centuries, our species has been watching this play performed using the tools of vision – we take various forms of radiation, like light, that leave the location of the play and travel to us in the audience. We interpret these observations, be they in light, or electrically charged particles, or even elusive particles called “neutrinos”, and learn something about the play. But detecting gravitational waves from the play is a whole new game, akin to shutting your eyes, pressing your ears against the side of the stage, and listening.
Where before we saw the play unfold with our eyes, with our ears we detect subtle things we never noticed before; we can hear Hamlet sneak up on Claudius as his uncle-father, believing that he is alone, kneels to confess his crimes; we hear the scuffle as Hamlet stabs Polonius, mistaking him for Claudius hiding in the room with Ophelia, the wild frenzy of shuffling feet and the horrible thud as Polonius hits the floor, his fate sealed; we hear the bodies drop, one by one, as dueling and poison takes the remaining characters at the end of the play. Listening to the stage on which the whole play is acted tells us new things about the play and its performance; similarly, listening to ripples in space and time, the framework on which all cosmic events play out, tells us something new about the way the Universe is.
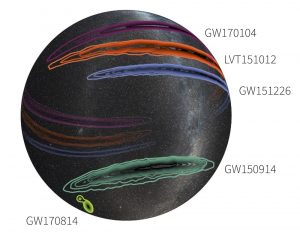
2017 brought even more stunning achievements in gravitational wave astronomy. LIGO again detected a merger and published their observations. Then, just a week ago, the LIGO and Virgo Collaborations announced the co-detection of the collision of two black holes. This led to a vast improvement to the ability, thanks to three detectors spread across the globe, to pinpoint where the collision happened in the sky. This is a remarkable achievement, in almost two years, from first evidence for gravitational waves to the operation of two independent collaborations and the co-detection of a black hole pair merger.
(GW170814: First triple-coincident observation of a black hole merger by the LIGO and Virgo detectors. Video c/o the Max Planck Institute)
The Nobel Committee recognized the breadth of this achievement by awarding the prize jointly to Kip Thorne,
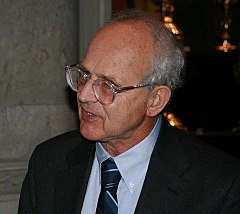
Rainer Weiss, born 1932 in Berlin, Germany, earned his PhD from MIT, where he is now a professor of physics. A refugee from the Nazi regime, he eventually settled with his family in the United States. He would go on to develop the ideas that allowed for laser interferometry to be utilized in the detection of gravitational waves. He did seminal work in the construction of early LIGO prototypes and was appointed the first spokesperson of the LIGO Scientific Collaboration. He receives a half-share of this year’s prize.
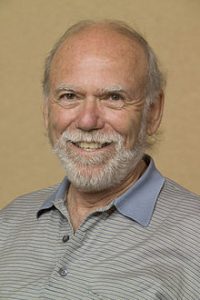
Barry Barish was born in 1936 and is a physicist at Caltech. While I know him most for his work on the development of the International Linear Collider project, it is clear that he has done exceptionally important work outside of collider physics. He served as the principal investigator of LIGO starting in 1994 and became the director of the project in 1997. He went on to create the much larger LIGO Scientific Collaboration, which incorporates more than just the LIGO instrument and its team of physicists and is clearly essential in making the current phase and pace of discovery possible.
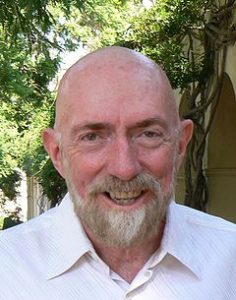
Thorne, born in 1940, is a theoretical physicist, also at Caltech, whose contributions to our modern understanding of gravity and ideas about how to detect gravitational waves have been essential to the founding and flourishing of the LIGO project. He is literally the co-author of the modern book on gravitation, and has even served as a writer and scientific advisor on films, such as “Interstellar.” His essential work on the physics of gravitational wave, and what would be required to detect them, was a key driver in the designs of the laser interferometers used by LIGO and Virgo (and soon, others).
All three are members of the LIGO-Virgo Collaboration.
While many more people were involved in making LIGO (and Virgo) a success, the recognition of these three is certainly meant to shed light on the importance of the first direct detection of gravitational waves and the opening of the era of gravitational wave astronomy, a new field of science.
Want more? I like this cool animated short video explaining LIGO and gravitational waves:
(c/o Phd Comics)
[1] A footnote is important here. I originally wrote this story in 2016, when I was hopeful that, despite coming after the official deadline for nominations, the LIGO result might anyway have garnered a Nobel in the same year as the announcement of the detection. At the time, founding LIGO physicist Ron Drever was still alive. Sadly, he passed away on March 7, 2017, and as the Nobel Prize cannot be awarded posthumously his contributions can never be honored at this level on the international stage. Nonetheless, his contributions to making this achievement possible have been remarkable and are worthy of praise, whether there is a Nobel Prize in a glass case or not. You can read Drever’s obituary in the New York Times.
[2] Goldstein, D. B. (2010). IS-GPS-800A GLOBAL POSITIONING SYSTEM WING ( GPSW ) SYSTEMS ENGINEERING & INTEGRATION INTERFACE SPECIFICATION IS-GPS-800 Revision A Navstar GPS Space Segment / User Segment L1C Interface, (June).