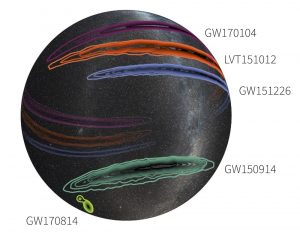
Sometimes, scientific fields move fast. They move so fast, even three authors working with a really responsive and excellent publisher who has fully embraced “print-on-demand” as a business model cannot keep up. Such is the reality of the new astronomy, gravitational wave astronomy. The LIGO, and then the VIRGO, instruments have worked so spectacularly well in the last two years (and are operated by such an effective team of scientists and engineers) that results from these instruments out-paced our ability to incorporate their discoveries fully into our writing. In a later edition of “Reality in the Shadows,” we’ll of course try to capture the full picture of the early period of this new astronomy. But for this post, it’s sufficient to have a look at something that just didn’t make it into our book: colliding neutron stars.
The Biggest Atom
In a sense, neutron stars are the biggest atoms in the universe. They are actually the corpses of dead stars. If a star is heavy enough (8-20 times the whole mass of our sun, or 8-20 “solar masses”) that its core, upon collapsing during one of its final stages of nuclear fusion, exceeds a mass of about 1.4 solar masses, then such a star can become a neutron star. This minimum core mass is known as the “Chandrasekhar Limit”. Should the core be as heavy as 3 times our sun (or heavier), then a much more interesting fate awaits – a black hole. For now, let us focus on a neutron star.
https://www.youtube.com/watch?v=RrMvUL8HFlM
To understand a neutron star, let us think about atoms. Atoms are composed of electrons bound to a central nucleus. The nucleus is composed of at least 1 proton and some number of neutrons. Protons are positively electrically charged, and cause the force of attraction that binds the electrons to the atom. The neutrons and protons bind to each other through the strong nuclear force, a force so strong it overcomes the mutual electric repulsion of the protons in a nucleus like that of Helium (or any element heavier than Helium).
At the kinds of pressures and temperatures present in a living star, the core of the star is capable of facilitating nuclear fusion: nearby nuclei are able to approach so close to each other that they merge, releasing energy (in the form of particles) in the process. The fusion process is an important counterbalance to the other major force at work in stars: gravity. Gravity tends to make the matter further out attracted to all the matter further in, close to the center of the star, which tends to collapse a star. But fusion causes an outward pressure (due to the kinetic energy of core particles and all the radiation particles from fusion), pushing back against collapse. So long as fusion can continue at a high enough rate, gravity can be balanced.
A dying star is in a state where it is not capable of fusing enough to resist its own collapse under gravity. And so the final act of a star begins. It collapses. During the collapse, the layers of the star outside the core are blown off. For a star like our own sun, the exposed core is left to glow as a white “cinder” – a white dwarf. No further collapse occurs.
But a white dwarf (a hot stellar core) that exceeds the Chandrasekhar Limit in mass will continue to collapse. The gravitational pressure is so great that electrons are crushed into the protons, merging through weak nuclear interactions to create neutrons and neutrinos (we discuss neutrinos in the chapter, “What the Heck’s the Higgs – Part 2”). Suddenly, where once there were a whole lot of protons and neutrons in the stellar core, there is an overabundance of neutrons. It’s as if a giant atomic nucleus has suddenly been forged from the collapsing core of a dying star.
Stopping the Collapse
You’d think this collapse would continue unabated, but this is only true if the stellar core is at least 3 solar masses in scale. Between 1.4 and 3 solar masses, a new balance is achieved. Neutrons, like all other subatomic particles, are really waves, not solid objects. Waves have to obey certain conditions when they are compressed into a space. For instance, think about music from an organ pipe. Sound is the result of the compression and relaxation of air, causing over-dense and under-dense regions. These are “sound waves.” For an organ pipe of a certain length – say, 3 yards long – the kinds of sound waves that are allowed are not unlimited. Only a certain multiple of a fundamental wave length can fit in the pipe – these are the fundamental note and its associated harmonics.
Similarly, when you compress neutrons too closely, since they are really waves then only certain lengths of those waves are allowed in the volume of the stellar core. This constrains the core to collapse only to a certain size… about 20 kilometers wide, but no smaller. You can think of what happens as if the neutrons exert a kind of “quantum pressure” that prevents the star’s collapse, but there is no pressure in the sense we would conceive in the macroscopic world (force per unit area). What’s really going on is that the neutrons have to have certain allowed speeds (momenta), and this prevents the further collapse of the star. The neutrons cannot have zero speed, which is what would be required to get them infinitesimally close together.
A stellar car alarm
Neutron stars are strange beasts. When formed by the collapse of a star, they are often born spinning very fast. This is because the collapse is never perfectly uniform from all directions, and the stellar core is highly turbulent during the collapse. The left over momentum from this process appears as spin in the neutron star. The stars have such huge magnetic fields when they are formed that the spinning magnetic field whips surrounding stellar debris into an electromagnetic frenzy, causing x-rays to be emitted from the hot remnants of the star’s atmosphere.
The energy of all of this spinning and x-rays is ridiculous; you don’t want to be anywhere near a neutron star when it’s born. It’s like somebody just set off the loudest, brightest, and deadliest car alarm in the universe. What kind of neighborhood would that be?
So far, I’ve talked as if the star was sitting in space all by itself. But stars are not only born, and don’t only die, alone in the cosmos. Most stars have a companion (some have more than one!). So it’s not uncommon that there may be pairs of neutron stars orbiting each other in the universe. Such orbiting pairs should emit gravitational waves – ripples in spacetime itself. If the stars close on one another as they dance together over millions of years, the waves get more intense. The waves are most intense just before and as the neutron stars collide and merge. It was, in fact, this kind of process that LIGO and VIRGO detected back in August of 2017.
A cosmic multimedia extravaganza
What was particularly remarkable about this neutron star merger, 130 million light-years from earth, is that LIGO and VIRGO were not the only telescopes that observed it. Almost 100 different kinds of telescopes – visible light, ultraviolet light, infrared light, x-rays, and gamma rays – across the globe and in orbit around the earth were able to see this event. This is what is known as “multimessenger astronomy” – using all the ways an event can be recorded to gather information about that event. LIGO and VIRGO captured the “sound” of the collision by observing the squashing and stretching of spacetime. Light-based telescopes recorded video of the event. This was a cataclysmic multimedia event.
We were not able to capture this incredible moment for astronomy in our book, but in talking about the black hole mergers that LIGO and VIRGO observed we hinted that such observations of neutron stars could be possible. How fortunate we are to live in a time when even authors in a modern publishing environment cannot keep up with the pace of discovery in the universe.
About “Reality in the Shadows”
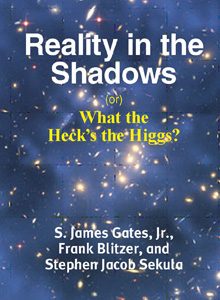
“Reality in the Shadows (or) What the Heck’s the Higgs” by S. James Gates Jr., Frank Blitzer, and Stephen Jacob Sekula. Available from Amazon.com, Barnes and Noble, Books a Million!, YBK Publishers, and other book retailers. $26.95.
Description:
In recent years, we have learned that the commonplace materials described by the scientific findings we have so far discovered portray but a tiny piece of a much greater universe–a universe ruled by vast mysteries that appear to shape reality at both its largest and smallest sizes. That universe is now coming to be better understood. You will see how this has happened and how the shadows in the unknown slowly continue to be lit and identified.
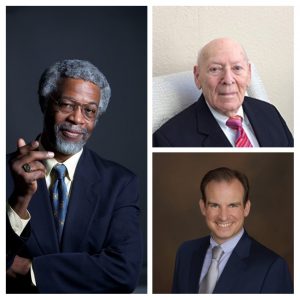
Reality in the Shadows is a chronicle of the men and women who cast light on these mysteries of our existence, a look into some of the brilliant ideas that they presented, and a longer look at the new and even greater mysteries of the cosmos that now cry for scientific explanation. It is also an opportunity to become familiar with a now-famous particle– the Higgs Boson–that is both a telling-out of some very old questions and the beginning for hundreds of new and yet-to-be-answered ones.
This book is written for the lay person–someone very interested in this subject, who may not have had a lot of technical preparation. It was prepared to make the material as engagingly easy to read as possible and provides many analogies and explanations.