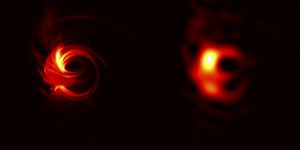
In our book, “Reality in the Shadows,” we devote an entire chapter to the phenomenon of the black hole (“A Shadow Where No Light Shines“). We dealt in things that are known – for instance, that black holes exist and that they can be detected using their effects on the surrounding space and matter – and things that are not known for certain – the mathematics needed to fully describe a black hole, for instance. Black holes are a deep dive. They represent the mass of at least one stellar core compressed into a volume smaller than the nucleus of an atom. Whereas neutron stars are like nature’s largest atomic nucleus, black holes are nature’s heaviest, but smallest, atomic nucleus. This makes them a challenge to modern physics. In a black hole, gravity is extremely strong… but so are the other forces of natures, those described by quantum physics. Yet no evidence-verified union of gravity and quantum physics exists. That makes black holes an excellent candidate to learn what we have not yet learned about places in the universe where gravity and quantum forces are both strong.
One of the exciting things that we didn’t get to include in the book, because it was not yet concluded as of publication, is an ongoing attempt to “photograph” the event horizon of the super-massive black hole at the center of the Milky Way galaxy, our home galaxy. In this essay, I’ll take a look at this effort and give you some ideas about just how big that black hole is, and why it might be possible to photograph it by tuning into it using radio waves.
Where no light shines
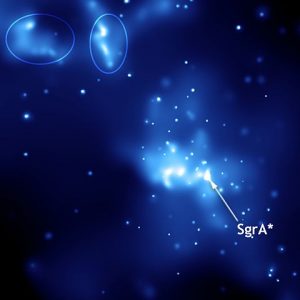
Light cannot escape the event horizon of a black hole. Spacetime is so warped by the mass at the center of the black hole that there is a point beyond which, as one approaches the center, no light wave sent backward away from the center can ever escape. Each black hole’s event horizon, the artificial boundary past which the black hole is black, is expected to be related directly to the mass inside the black hole. The super-massive black hole at the center of our galaxy, called “Sagittarius A*,” is estimated to contain a mass of 4,000,000 suns. From this information, we can estimate the size of its event horizon.
There is a simple mathematical relationship between mass and event horizon, given as follows:
This is also known as the Schwarzschild Radius, the distance within which if you compress a mass, M, it will form a black hole. For Sagittarius A*, this radius is about 10 billion meters. G is Newton’s Gravitational Constant, and c is the speed of light in empty space. The only variables in this equation are the mass and the radius; one controls the other.
Treating the black hole as a sphere of radius, R, then we would expect the event horizon to look like a dark region (warping the space-time around it extremely strongly) with a diameter of 20 billion meters. That sounds big, but how big is that, really, when you take into account our distance from the center of the Milky Way?
Far from downtown
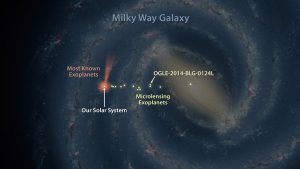
If the center of the Milky Way is “downtown,” then earth inhabits a far-flung suburb of our galactic city. We are, in fact, about 26,000 light-years from Sagittarius A*… that means it takes like 26,000 years to make the journey from Sagittarius A* to earth. Since we can easily see the center of the Milky Way with radio (which helps punch through the dust between us and the center of the galaxy), we are in fact seeing the center as it appeared 26,000 years ago… that predates written human history. In fact, at the time that the light we see now left Sagittarius A*, humans had not even developed agriculture.
So how far is 26,000 light-years? It’s about 250 billion billion meters, written in scientific notation as 2.5 with 20 zeros after it, or .
How does that compare to the event horizon? In scientific notation, the event horizon of Sagittarius A* has a diameter of . We see that the distance to Sagittarius A* is almost 10 billion times bigger than the event horizon of the black hole. We can use an old trick from high school geometry to convert this into a more useful number: the angle “subtended” by the event horizon, from earth’s perspective. Thing of a pizza that has been sliced into 8 equal-angle pieces. Each piece fills 1/8 of the full 360 degrees of the circle, and so each piece can be said to “subtend” an angle of
So what angle does the event horizon of Sagittarius A* subtend? An approximate formula, which for these sizes and distances is very accurate, is just
where R is the event horizon radius and D is the distance from earth to Sagittarius A*. The answer is then:
This is a very small angle. To give you a sense of scale, Pluto, which can barely be seen with an 11-inch reflecting telescope and is considered a delightful challenge for an amateur astronomer to spot, subtends an angle of . That’s almost 10 million times bigger than the angle subtended by Sagittarius A*. As another point of comparison, the only other star in the universe whose surface we’ve captured an image of is Antares, a red super-giant star in our stellar neighborhood. Antares subtends an angle of about
. Again, that is still more than 10,000 times bigger than the angle subtended by Sagittarius A*!
To put this in final perspective, seeing the event horizon of Sagittarius A* would be like trying to spot a single bacterium in a petri dish sitting on a bench in a building in downtown Dallas, but doing so from my home in the suburbs, about 40km away. A single bacterium, at that distance, subtends about the same angle as does Sagittarius A* when viewed from earth.
So how is it that astronomers have been trying to capture the image of something so tiny, so far from our planet?
Whole-earth telescope
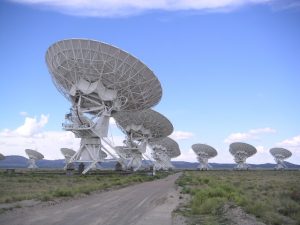
Radio telescopes are located across the globe. By combining them together to observe Sagittarius A*, it is possible to obtain an angular resolution – a precision of seeing – that is sufficient to achieve an image like that shown at the top of this post. That is remarkable. In effect, one is using the whole earth as the base of the measurement, ganging together many radio telescopes to form one large “radio eye” capable of resolving an object the size of the Sagittarius A* event horizon. To make precise observations, the radio astronomers are using a key property of light waves – interference – to boost the sensitivity of the measurement to very small angles. This is the same property of light that was used to observe gravitational waves, which are ripples in space-time that expand and compress the earth a distance about a million times smaller than an atomic nucleus. This will certainly wind up being one of the most impressive uses of a radio telescope array, should it prove successful.
And what have they seen? The results are not in [1]. We know the astronomy team is working on the data, cleaning up the noise that inevitably pollutes any image taken with any instrument. Results are certainly expected in the next year. Perhaps in some later edition of “Reality in the Shadows,” we’ll be able to speak of photos of the event horizon of a black hole.
What will we see? What will we learn about gravity and how it distorts light and matter just outside such a massive black hole? These are exciting times! We have seen black holes colliding and rippling the fabric of space-time, bringing messages to us from the event horizons of newly formed black holes. Soon, we will have a radio image of the event horizon of a black hole. I wonder what can be learned from all of this?
The Maelstrom
On April 10, 2019, the National Science Foundation and the Event Horizon Telescope collaboration held a media event [2] announcing the first-ever picture of the region around a black hole – specifically, the supermassive at the center of the galaxy M87! It was a remarkable image, and implied a mass of 6.5 billion suns. This is even larger than our own supermassive black hole is expected to be, making it possible to image in a neighboring galaxy.
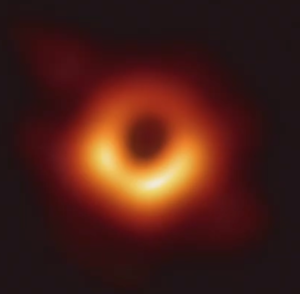
According to the publication from the Event Horizon Telescope collaboration, the M87 supermassive subtends a scale more like . This is more akin to the challenge faced in generating an image of the surface of the star Antares, but still at least 10 times more difficult… and in another galaxy!
As was said at the press conference, this is just the beginning. While the picture reveals features so-far consistent with the General Theory of Space and Time (Relativity), this becomes a new treasure trove for investigating the interaction of space, time, energy, and matter.
To see the time evolution of the image, especially checkout their paper in The Astrophysical Journal Letters [3].
References
[2] https://www.youtube.com/watch?v=lnJi0Jy692w
[3] https://iopscience.iop.org/journal/2041-8205/page/Focus_on_EHT
About “Reality in the Shadows”
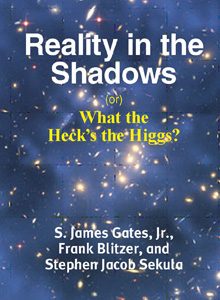
“Reality in the Shadows (or) What the Heck’s the Higgs” by S. James Gates Jr., Frank Blitzer, and Stephen Jacob Sekula. Available from Amazon.com, Barnes and Noble, Books a Million!, YBK Publishers, and other book retailers. $26.95.
Description:
In recent years, we have learned that the commonplace materials described by the scientific findings we have so far discovered portray but a tiny piece of a much greater universe–a universe ruled by vast mysteries that appear to shape reality at both its largest and smallest sizes. That universe is now coming to be better understood. You will see how this has happened and how the shadows in the unknown slowly continue to be lit and identified.
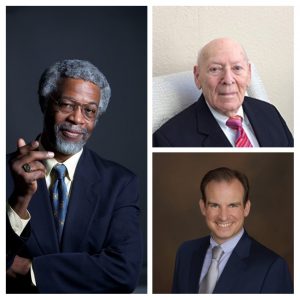
Reality in the Shadows is a chronicle of the men and women who cast light on these mysteries of our existence, a look into some of the brilliant ideas that they presented, and a longer look at the new and even greater mysteries of the cosmos that now cry for scientific explanation. It is also an opportunity to become familiar with a now-famous particle– the Higgs Boson–that is both a telling-out of some very old questions and the beginning for hundreds of new and yet-to-be-answered ones.
This book is written for the lay person–someone very interested in this subject, who may not have had a lot of technical preparation. It was prepared to make the material as engagingly easy to read as possible and provides many analogies and explanations.