My present focus is on the search for the nature of dark matter and on methods for early detection of galactic supernovas. I am a member of the PICO dark matter bubble chamber collaboration as well as the HALO (Helium and Lead Observatory) supernova neutrino detector collaboration.
Prior to 2023, my research was primarily in collider physics. My focus then was on interactions of the Higgs particle with heavy quarks, such as the bottom quark, and with itself (the so-called “Higgs self-coupling”). This work was done using the ATLAS Experiment at the Large Hadron Collider. I was also exploring the use of charm quarks as a probe of the structure of the proton, neutron, and whole nuclei. This was being done as part of the ATHENA proto-collaboration in preparation for the Electron-Ion Collider.
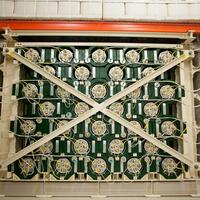
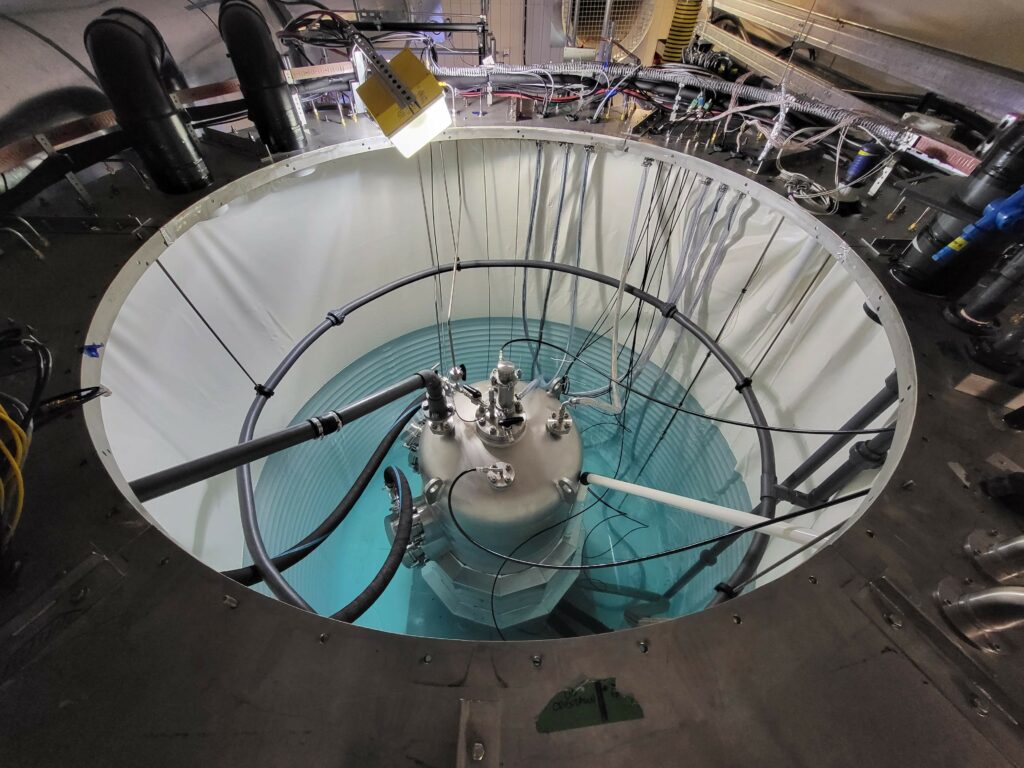
My current research focus:
- Construction, operations, and monitoring of the PICO-40L detector for a 2022-2024 data-taking campaign.
- HALO data processing pipeline development, operating on the SNOLAB Nearline computing system.
Contents
Stories of Science
Key Contributions to the Field
- 2018: Discovery of the decay of the Higgs particle to a pair of bottom quarks
- Largest decay mode of the Higgs. Predicted in the 1960s-1970s. One of the most challenging measurements at the Large Hadron Collider (limited by systematic uncertainties).
- My contributions: analysis framework; reduction in systematic uncertainty due to limited simulation sample sizes; modeling and reduction of systematic uncertainty from top-quark background process.
- 2012-2013: Measurement of the Spin-Parity quantum numbers of the particle discovered in 2012 with a mass of 126 GeV
- Established the Higgs particle hypothesis as the leading explanation of the newly discovered particle.
- My contributions: utilization of multivariate machine-learning approaches to improve pair-wise hypotheses testing and discrimination
- 2008: Discovery of the bottomonium ground state
- Predicted to exist in 1977, but eluded detection for 31 years.
- My contributions: led the data-taking campaign that made the measurement possible.
Past Research
Understanding the Higgs Particle
The discovery of the Higgs Particle in 2012 began a 30-year program to map its properties. One of the key properties of this rare particle is the degree to which its mass-energy is converted into a pair of bottom quarks – the second-heaviest building block of nature. The research team I lead at SMU, part of the larger SMU ATLAS research group, is focused on the measurement of this process. We helped to discover this process in 2018 and contributed to thre first comprehensive measurement from the ATLAS Experiment using all available data.
My effort to understand the Higgs and bottom quark interaction was largely focused on the case where the Higgs particle is produced alongside a heavy weak boson (the W or Z particle), but in addition I contributed to the exploration of high-momentum Higgs production from gluon fusion and vector-boson fusion. Especially in these latter cases the emphasis was on applying advanced computational techniques to better use quark and gluon jet structure for Higgs discrimination.
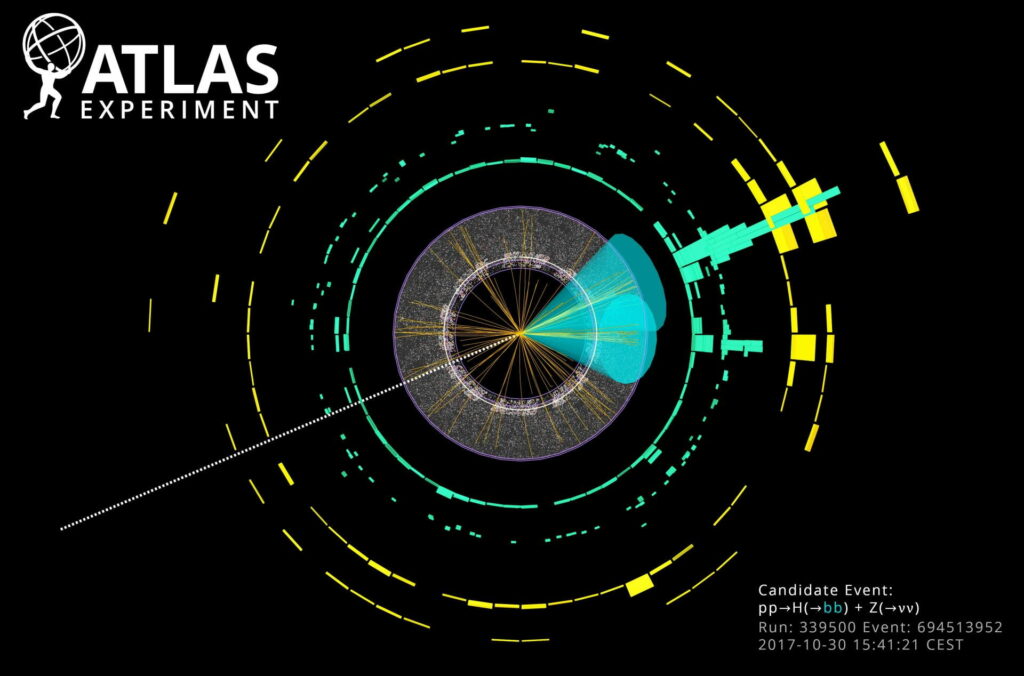
Once we in the community definitively observed the Higgs and bottom quark interaction, my attention began to turn to using this process to make an even more fundamental discovery.
Searching for the Higgs Force Field

The Higgs particle was a direct consequence of the mechanism that gives rise to fundamental mass. The discovery of this particle was a key step in understanding that mechanism. But the most fundamental part of the mechanism was the introduction of a scalar gauge field, ϕ, that gives rise to the Higgs particle. The field is the fundamental prize in the Brout-Englert-Higgs Mechanism, and yet it has eluded direct detection.
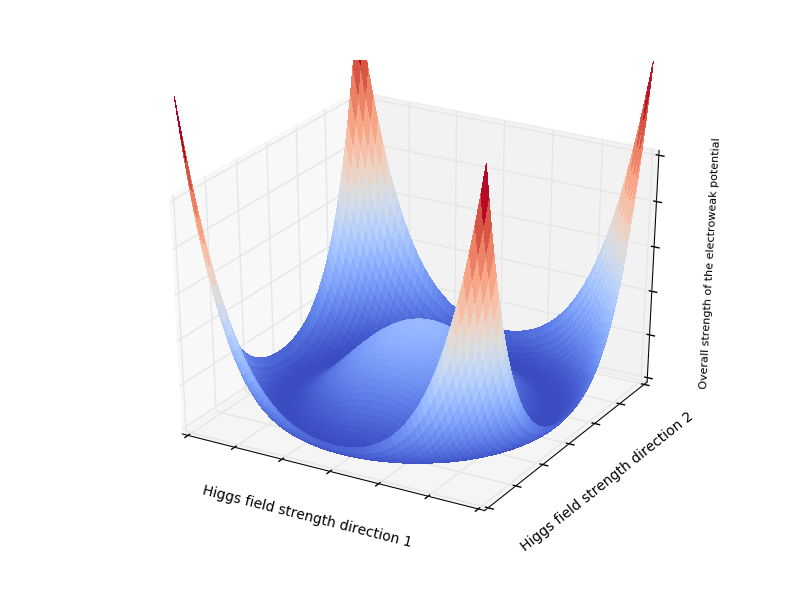
A key way to probe the structure of the Higgs field directly is to observe coincident production of a pair of Higgs particles at the Large Hadron Collider. This process became a key target of our field beginning in the 2015-2018 “Run 2” era of the LHC. One high-sensitivity probe of this process is the use of the Higgs and bottom quark interaction that we directly observed starting in 2018.
In order to secure proton-proton collisions with these interactions, we need real-time data collection algorithms (“triggers”) that store the data for later analysis. The ATLAS Experiment can only record 2000 of the 40 million events produced by the LHC each second. I co-led the ATLAS b-jet Trigger Signature Group tasked with securing the collection of bottom-quark jets, a key signature of the Higgs decay to bottom quarks. My group at SMU worked in this area of the trigger, in part to develop new ways to select these events in data at the moment of the proton-proton collisions that produce them.
My most recent efforts here were focused on evolving new strategies for this work in ATLAS Run 3 data campaign. My final Ph.D. student on the ATLAS Experiment developed higher-dimensional mathematical methods to understand two-Higgs production using the more rare Vector-Boson Fusion-induced Higgs production mechanism. That production process provides a more unique topology (e.g for event triggering) but also fewer expected events in the currently available data samples. Run 3 was expected to greatly increase the potential for such rare production mechanisms. I wish all of my colleagues in the LHC communty a marvelous journey into this space of measurements.
Proton Tomography
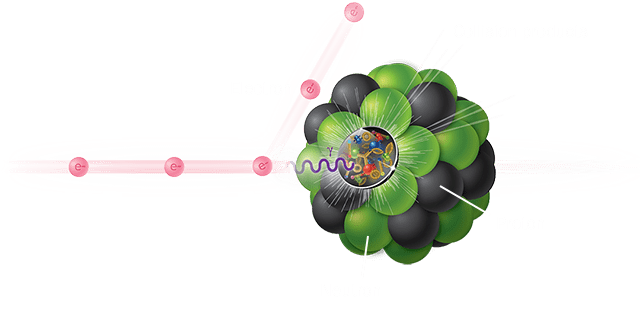
The planned Electron-Ion Collider (EIC), to be located at Brookhaven National Laboratory in Upton, NY, will usher in a new era of precision interrogation of nuclear substructure. I was particularly interested in the role that the heavier quarks – strange, charm, and bottom – play in the structure of the proton. In the coming 20 years, I am excited for my colleagues to complete the work we envisioned: using particle jets as a precision probe of the contribution to spin and overall structure that these quarks provide to the everyday proton.
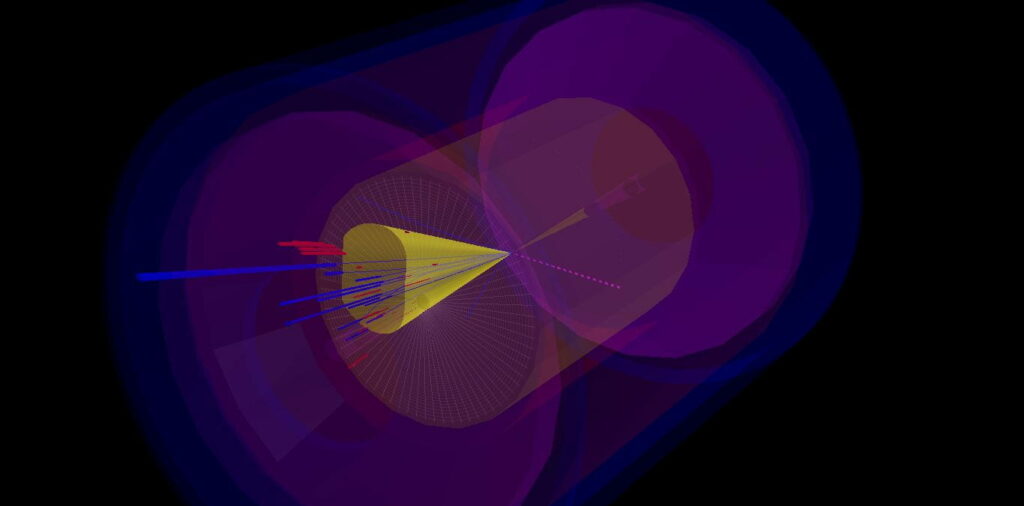
Detailed knowledge of the proton is essential to the LHC program in the 2030s. The EIC could not be better timed, and aims for operation to begin at the end of the 2020s and early 2030s. The lessons from the LHC will enable the LHC to fully realize its precision science goals. The hard work to define and utilize particle jets at the LHC will inform the use of jets to infer proton structure at the EIC. These complementary programs provide many new opportunities for the community.