[This post was inspired by a comment in an article on PhysOrg, http://www.physorg.com/news/2011-09-cern-faster-than-light-particle.html. Thanks to Randy Scalise for bringing it to my attention.]
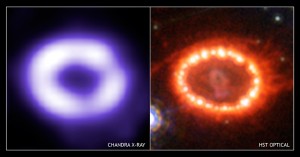
In 1987, a distant star exploded. Here on Earth, it was named “SN1987a” – Supernova 1987a. Here are some basic facts about SN1987a: it occurred (51.4 +/- 1.2) kiloparsecs from Earth, corresponding to a distance in meters of (1.586 +/- 0.037)x1021 m, and we saw the visible light from the event beginning on Feb. 23, 1987.[1] [2]
Light travels at a finite speed. In the vacuum – completely empty space – that speed is 299,792,458 m/s (meters per second). The uncertainty on that speed is in the last digit, which represents a precision far greater than our knowledge of the distance to SN1987a. Therefore, in all future calculations the uncertainty on this speed will be ignored and numbers will be assumed to have uncertainties dominated by the distance measurement.
Once the light from the supernova collapse and subsequent explosion escapes the star, the time required to travel from SN1987a to Earth is (5.290 +/- 0.0736)x1012s. There are about 3.153×107 seconds per year (fast fact: you can very closely approximate the number of seconds in a year by multiplying the number π, 3.14159…, times 107s. That gives you 3.14×107s, which we see is extremely close to the correct number). If we convert to years, the light from SN1987a required (167,800 +/- 3900) years to travel to Earth.
A recent preliminary, un-reviewed, unpublished, and unconfirmed result from the OPERA Collaboration suggests that neutrinos travel faster than light [3]. Specifically, within the framework of their measurement they find that the speed that muon neutrinos travel through the Earth from CERN to their experiment is (299,799,893 +/- 1,230) m/s.
Supernova collapse is known to lead to the production of neutrinos; in fact, neutrinos from SN1987a were detected by multiple neutrino experiments that were operating in the late 1980s. Based on the difference in speed between light and muon neutrinos, where muon neutrinos are measured by OPERA to travel at a speed EXCEEDING that of light in vacuum, let’s see when we would have expected the supernova-produced neutrinos to arrive at Earth.
Before we proceed, let’s note that we have a potential problem with this calculation – the uncertainty on the distance to SN1987a is HUGE. Our uncertainty on how long light took to travel from SN1987a to Earth has an uncertainty of 3900 years – that covers the entire period of human development back to ancient civilizations such as China and Egypt. Instead, let’s calculate the RATIO of travel times of light and neutrinos. We can then apply this ratio to any time period and evaluate the relative arrival times of neutrinos and light.
The OPERA paper actually provides this number. The ratio of travel times between neutrinos and light is:
(v-c)/v = (2.48 +/- 0.41)x10-5
which means that the neutrinos arrive 0.00248% faster than light. What does that mean for SN1987a?
If we take the light travel time to be 167,800 years (exactly), then in that same time neutrinos take 167,796 years to reach Earth. That’s 4 years earlier than the light. What if, instead, the travel time was 3900 years less (one standard deviation DOWN). Then the travel time of light is 163,900 years and for neutrinos it is 163,896 years – again, 4 years earlier.
So the time difference, regardless of the ACTUAL time it took light to travel, is about 4 years. One would not expect neutrinos to accompany the light when the light reached Earth in 1987.
What was observed?
Here is the summary from the Wikipedia article on SN1987a:
Approximately three hours before the visible light from SN 1987A reached the Earth, a burst of neutrinos was observed at three separate neutrino observatories. This is likely due to neutrino emission (which occurs simultaneously with core collapse) preceding the emission of visible light (which occurs only after the shock wave reaches the stellar surface). At 7:35 a.m. Universal time, Kamiokande II detected 11 antineutrinos, IMB 8 antineutrinos and Baksan 5 antineutrinos, in a burst lasting less than 13 seconds. Approximately three hours earlier, the Mont Blanc liquid scintillator detected a five-neutrino burst, but this is generally not believed to be associated with SN 1987A. [4]
Neutrino interactions in the detectors were observed to increase in rate just before the light reached the Earth. This was consistent with the physics noted above; neutrinos are essentially free to leave the star once it collapses since the material density in the star is not sufficient to completely prevent neutrinos from leaving. Light, however, is trapped in the collapse until the blast wave reaches the surface of the star; this is at a later time than the nuclear reactions that produced neutrinos. So even though light travels slightly faster than neutrinos (due to the neutrinos’ small but non-zero masses), the light didn’t catch up before reaching Earth and the neutrinos arrived about 3 hours before the light.
So the time difference was just 3 hours, not 4 years. Of course, nobody was looking for neutrinos from SN1987a 4 years before it happened, but the fact that a burst of neutrinos was observed just hours before the light is evidence that the neutrinos were not too far ahead of the light. Calculations of the neutrino and light arrival times within the framework of core-collapse supernova modeling suggest that this time difference (neutrinos leading light) is not a surprise, given that neutrinos escape before light escapes the supernova.
Some criticisms of this calculation
- This calculation assumed that the neutrinos produced by supernova are the same as those studied by OPERA. OPERA studies muon neutrinos. The neutrino experiments which detected neutrinos from SN1987a were sensitive to electron neutrinos. So all we can really say is that electron neutrinos arrived just hours before light. Muon neutrinos may have also been produced directly by SN1987a, or produced by neutrino mixing between the explosion and the time the neutrinos reached Earth. One could argue, therefore, that perhaps the undetected muon neutrinos arrived much earlier.
- Nobody was looking for muon neutrinos from space/supernovas in 1983. That’s a hole in the argument, given that the calculation suggests the muon neutrinos would arrive 4 years before the light.
A comment on item #1: there is no evidence that electron neutrinos are so different from muon neutrinos. One would have to gather such evidence. In the meantime, one would have to postulate a mysterious and VAST difference in the speeds of electron and muon neutrinos.
Another comment: neutrino mixing is easily explained if neutrinos have mass. Mass prevents a particle from traveling at the speed of light in vacuum. If the OPERA result is correct, very little makes sense anymore regarding the Theory of Relativity, which has withstood precision tests for about a half-century. Certainly, it only takes one confirmed and reproducible measurement to bring a scientific theory into question. The OPERA result is neither confirmed nor even reproduced at this point. It’s not even published.
Conclusions
The neutrino is a mysterious particle. But so far, it hasn’t been so mysterious as OPERA would suggest. Data from SN1987a suggests that electron-type neutrinos arrived just hours before light, consistent with the different interactions neutrinos and light would suffer in the environment of a core-collapse supernova. This contradicts the expectation from OPERA, albeit that measurement applies to muon-type neutrinos.
Personally, I’m not holding my breath for this result. I’ll bet anybody $10 it’s wrong. And if instead I am wrong, I will pay up with a smile on my face and joy in my heart.
[1] http://heritage.stsci.edu/1999/04/fast_facts.html
[2] Panangia, N. “Distance to SN 1987 A and the LMC.” New Views of the Magellanic Clouds, IAU Symposium #190, Edited by Y.-H. Chu, N. Suntzeff, J. Hesser, & D. Bohlender. http://adsabs.harvard.edu/full/1999IAUS..190..549P
[3] http://arxiv.org/abs/1109.4897
[4] http://en.wikipedia.org/wiki/SN_1987A
One thought on “Head start”
I found you through SMU Physics on Facebook. I could not find an email address, so, here goes:
I saw that you are involved in the ATLAS collaboration. I wanted to apprise you of two projects in Public Distributed Computing which are part of the efforts at CERN for the LHC.
http://lhcathomeclassic.cern.ch/sixtrack/, or LHC@home/Sixtrack is a project running on BOINC software which aids in the tuning of magnets to reduce wasted time and effort in the beamline.
http://lhcathome2.cern.ch/test4theory/ or LHC@home 2.0 simulates collision events.
These and other research projects running on software from http://boinc.berkeley.edu are aimed at personal computer users. The most famous and the original project was SET@home.
We earnestly need to attract as many people with as many personal computers as possible to scale up these two projects as CERN gets ready for a 202 rebuild.
If you could help us by spreading the word through your department it would be greatly appreciated.
If you are not familiar with the projects, you can conveniently access them together at
http://lhcathome.cern.ch/